Waunita Hot Springs Geothermal Area
Area Overview
Geothermal Area Profile |
Location: | Colorado | |
Exploration Region: | Rio Grande Rift | |
GEA Development Phase: | ||
Coordinates: | 38.4700°, -106.5350° | |
Resource Estimate |
Mean Reservoir Temp: | ||
Estimated Reservoir Volume: | ||
Mean Capacity: | ||
USGS Mean Reservoir Temp: | 120°C 393.15 K 248 °F 707.67 °R |
[1] |
USGS Estimated Reservoir Volume: | 2 km³ | [1] |
USGS Mean Capacity: | 12 MW | [1] |
- "<br> Waunita Hot Springs is located near the border of Gunnison and Saguache counties in south-central Colorado, USA, approximately 25 miles east of the town of Gunnison. The springs, at 9,000 feet in elevation, reside in a high intermontane valley 10 miles west of the Continental Divide at Monarch Pass. The hot springs actually consist of two groups of springs, dubbed the Upper and Lower Waunita Hot Springs, roughly half a mile apart. The springs themselves are on private land, but are surrounded by state and federal lands (Figure 1). The hot springs are accessible from County Road 887, a dirt track. U.S. Highway 50 to the south is the nearest paved roadway. Tomichi Dome, a sizable accumulation of rhyolite flows to the southwest of the hot springs, is the most prominent nearby topographic feature." cannot be used as a page name in this wiki.
- The given value was not understood.
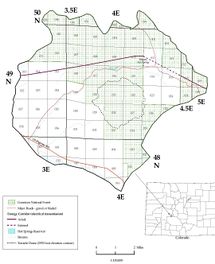
Waunita Hot Springs is located near the border of Gunnison and Saguache counties in south-central Colorado, USA, approximately 25 miles east of the town of Gunnison. The springs, at 9,000 feet in elevation, reside in a high intermontane valley 10 miles west of the Continental Divide at Monarch Pass. The hot springs actually consist of two groups of springs, dubbed the Upper and Lower Waunita Hot Springs, roughly half a mile apart. The springs themselves are on private land, but are surrounded by state and federal lands (Figure 1). The hot springs are accessible from County Road 887, a dirt track. U.S. Highway 50 to the south is the nearest paved roadway. Tomichi Dome, a sizable accumulation of rhyolite flows to the southwest of the hot springs, is the most prominent nearby topographic feature.
History and Infrastructure
Operating Power Plants: 0 |
No geothermal plants listed. |
Add a new Operating Power Plant |
Developing Power Projects: 0 |
No geothermal projects listed. |
Add a new Developing Power Project |
Power Production Profile |
Gross Production Capacity: | ||
Net Production Capacity: | ||
Owners : | ||
Power Purchasers : | ||
Other Uses: | ||

The exploration party of John C. Fremont made use of the hot springs for bathing in the 1840s, marking the first time the springs were documented by Europeans. By 1882, Charles Elgin had built a hotel near the Upper Springs, to be followed by a bathhouse and pool fed by the thermal waters [4]. Since then, both springs have been under private ownership, with the Upper Springs and surrounding property consistently being used for recreational purposes. In 1962, the Pringle family purchased the property encompassing the Upper Springs and developed into its current use as a guest dude ranch and spa (Figure 2) [3].
Limited infrastructure exists in the resource area. County Road 887, a dirt track, offers the nearest vehicular access, with U.S. Highway 50 to the south representing the nearest paved roadway. A spur of the Arkansas River main line of the Denver and Rio Grande Western Railroad approaches Monarch Pass, 12 miles east of Waunita Hot Springs. The Colorado-Ute Electric Association cooperative and the U.S. Bureau of Reclamation (USBR) service electricity to the area, with115 and 230 kVe transmission lines owned by the USBR passing a few miles to the north of the hot springs [4].
Principal industries in the region include farming of hay and cool weather vegetables, raising stock (sheep, cattle, and horses) and logging. Some mining of copper, zinc, fluorite, lead, gold, silver, coal, and building materials also occurs. In the 1950s, there was some exploration for uranium toward Gunnison and to the southwest of Doylesville. Tourism has become a significant industry in the region, with resorts offering skiing, hunting, fishing, and dude ranching throughout the year [4].
Population in the area is minimal, with Gunnison and Saguache Counties having population densities of 4.7 and 1.9 person per square mile, respectively, as of 2010. This is well below the state average of 48.5 persons per square mile. The nearest population centers are the towns of Gunnison, with 5,854 persons, 25 miles to the west of Waunita Hot Springs, and Salida, with 5,236 persons, 30 miles to the east [5].
Regulatory and Environmental Issues
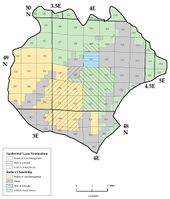
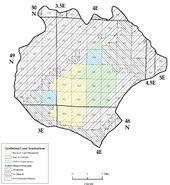
Exploration History
First Discovery Well |
Completion Date: | ||
Well Name: | ||
Location: | ||
Depth: | ||
Initial Flow Rate: | ||
Flow Test Comment: | ||
Initial Temperature: | ||
- "File:Aeromagnetic map.PNG|270px|thumb|right|Figure 5: Bouguer gravity map of study region '"`UNIQ--ref-0000000F-QINU`"']]
The first documented geophysical exploration work near Waunita Hot Springs was two groundnoise and microearthquake surveys, conducted in December 1971 and February 1972 by Senturion Sciences. One major groundnoise anomaly of 56 dB at a predominant frequency of 1 Hz was identified approximately 3,000 m NE of Waunita Hot Springs, which was attributed by Senturion Sciences to faulted, altered Paleozoic sediments '"`UNIQ--ref-00000011-QINU`"'. However, the data acquired are somewhat questionable as no base station was used to account for daily or longer term variations in background noise '"`UNIQ--ref-00000012-QINU`"'. Additionally, a relatively large number of microearthquakes were detected in the near vicinity of the hot springs, providing strong evidence for fracture permeability playing a prominent role in conducting geothermal fluids. Senturion Sciences interpreted the results of the microearthquake surveys as indicative of a radial fault system centered on Tomichi Dome '"`UNIQ--ref-00000013-QINU`"'.
In 1972, the U.S. Geological Survey (USGS) conducted coarse-scale (1:500,000) gravity surveys (Figure 5) and aeromagnetic (Figure 6) of Colorado which indicated the intersection of two prominent lineaments at Waunita Hot Springs. The hot springs also reside in the minimum of a N-S magnetic depression. However, the scale was too coarse to explicitly identify Tomichi Dome or other local features '"`UNIQ--ref-00000014-QINU`"''"`UNIQ--ref-00000015-QINU`"'.
The next round of exploration work was conducted in 1981. During this time, Henrichs Geoexploration Company conducted two phases of eletrical resistivity tomography around the Waunita Hot Springs and Tomichi Dome area. The first phase consisted of mapping and profile dipole-dipole surveys and Schlumberger soundings. The mapping dipole survey consisted of 103 stations over a 3 by 4 mile area using a transmitting dipole 7,000 feet in length and an orthogonal pair of 300 foot dipoles at each station. Injected currents of 6-8 amperes and a filtering frequency of 3.0 Hz were used. The profile dipole survey used dipole with a length of 200 feet and transmitting-receiving station separations of 1-9 dipole lengths. An injected current of 5 amperes and 3.0 Hz frequency was used. The Schlumberger soundings involved a transmitting dipole with length ranging from 500-2,500 feet for a 100-foot receiving dipole to 2,500-15,000 feet for a 500-foot receiving dipole. Injected currents of 2-6 amperes were used with a frequency of 0.05 Hz read as an averaged DC-coupled signal by the receiver. The second phase consisted only of dipole-dipole mapping, with a transmitting dipole length of 7,000 feet and 132 stations spread over several areas of interest identified in Phase I. All stations used an orthogonal pair of 300-500 foot dipoles. Injected currents of 2-8 amperes were used with frequencies of either 0.1 or 0.3 Hz to eliminate induced coupling effects. All measurements were obtained using a GEOEX Mark 4 induced polarization-resistivity system '"`UNIQ--ref-00000016-QINU`"'.
The resistivity surveys identified a low-resistivity zone approximately 7 km by 3.5 km in area extending NW to SE between Waunita Hot Springs and Black Sage Pass. Apparent resistivities in the zone ranged from 33-300 ohm-meters, with the lowest readings in synclinal Mesozoic sediments 2-3 km west of Black Sage Pass. Henrichs Geoexploration Company [1981] interpreted this zone as a 50 ohm unit of Mesozoic sediments over a 2000 ohm Precambrian crystalline basement. Contour mapping also suggested a possible resistivity low beneath the southern flank of Tomichi Dome; however, poor access limited coverage beneath the dome itself '"`UNIQ--ref-00000018-QINU`"'. It should be noted that these surveys were conducted prior to the advent of modern inversion techniques (and computing power) and, as such, a reanalysis of these data may prove fruitful in identifying or better characterizing anomalies potentially associated with geothermal reservoirs.
In addition to the geophysical data, in the summer of 1980, the Colorado Geologic Survey (CGS) analyzed 65 soil samples from the Waunita Hot Springs area for mercury concentrations as part of its geothermal resource assessment program. Samples were taken from a series of profile lines within an approximately 0.06 sq. mi area surrounding the hot springs (Figure 7). Additonally, several samples were taken approximately 1000 ft to the NE of the sample area to account for background soil mercury concentrations. Sample lines were designed to cross known nearby structural features as well as the near vicinity of the hot springs themselves. Soil mercury content ranged from 2-179 ppb in the analyzed sample, with a mean of 19.46 ppb and standard deviation of 31.76 ppb. Unfortunately, the survey results were inconclusive, with no identifiable pattern that would be indicative of thermal systems apart from those already known '"`UNIQ--ref-00000019-QINU`"'.
Eight thermal gradient boreholes were installed by AMAX Exploration, Inc. within a 3.6 km radius of Waunita Hot Springs, at an undetermined time prior to 1981 (Figure 8). All boreholes were drilled to 100 m depth except #3 and #7, which were completed to 88 and 82 m, respectively. Boring logs are of limited detail with regard to lithology, but generally indicate sequences of clay, shale, and sandstone, with some rhyolite in Boreholes #5 and #8 and schist in Borehole #6. The water table was encountered between 14 and 55 meters in most boreholes, though it is unknown if these boreholes were allowed to equilibrate first. Bottom hole temperatures for most ranged from 7.1ºC (in Borehole #7) to 14ºC (in Borehole #6); however, Borehole #3 exhibited an anomalously high bottom hole temperature of 47.5ºC, along with the highest calculated thermal gradients for any of the boreholes (Figure 9). Borehole #3 was the closest to the hot springs, located approximately 0.6 km to the southwest of the Upper Springs on the opposite side of County Road 887 '"`UNIQ--ref-0000001B-QINU`"'.
Most boreholes exhibited significant anisotropy in thermal gradients, except Boreholes #1 and #6, for which differential thermal gradient data was not available, and Borehole #8, which was the furthest away (~3.6 km) from the hot springs, on the opposite side of Tomichi Dome. Again, Borehole #3 exhibited the greatest anisotropy, with a reported temperature gradient of 1,080ºC/km between 34-40 m depth, and gradients of 340ºC/km and 247ºC/km immediately above and below, respectively '"`UNIQ--ref-0000001C-QINU`"'. This anisotropy in thermal gradient argues for a hydrothermal system dominated by by fracture or similar preferential flow in these anomalously high zones. As such, the utility of these boreholes in assessing deeper crustal heat flow and potential reservoir temperatures is severely limited. Averaging the temperature gradient from the surface to the bottom of the boreholes may provide a more reasonable estimate of the overall thermal flow for the resource area. These values range from 25 to 62ºC/km for all the boreholes, with 25ºC/km representing the average thermal gradient of the continental crust. The boreholes which exhibited the lowest average gradient were several kilometers from the hot springs and up-dip. None of them penetrated the Dakota Sandstone '"`UNIQ--ref-0000001D-QINU`"'. [[File:Heat Flow Map.PNG|270px|thumb|right|Figure 9: Heat flow contour map and temperature gradient borehole locations '"`UNIQ--ref-0000001E-QINU`"'" cannot be used as a page name in this wiki. - The given value was not understood.
The first documented geophysical exploration work near Waunita Hot Springs was two groundnoise and microearthquake surveys, conducted in December 1971 and February 1972 by Senturion Sciences. One major groundnoise anomaly of 56 dB at a predominant frequency of 1 Hz was identified approximately 3,000 m NE of Waunita Hot Springs, which was attributed by Senturion Sciences to faulted, altered Paleozoic sediments [8]. However, the data acquired are somewhat questionable as no base station was used to account for daily or longer term variations in background noise [9]. Additionally, a relatively large number of microearthquakes were detected in the near vicinity of the hot springs, providing strong evidence for fracture permeability playing a prominent role in conducting geothermal fluids. Senturion Sciences interpreted the results of the microearthquake surveys as indicative of a radial fault system centered on Tomichi Dome [8].
In 1972, the U.S. Geological Survey (USGS) conducted coarse-scale (1:500,000) gravity surveys (Figure 5) and aeromagnetic (Figure 6) of Colorado which indicated the intersection of two prominent lineaments at Waunita Hot Springs. The hot springs also reside in the minimum of a N-S magnetic depression. However, the scale was too coarse to explicitly identify Tomichi Dome or other local features [6][7].
The next round of exploration work was conducted in 1981. During this time, Henrichs Geoexploration Company conducted two phases of eletrical resistivity tomography around the Waunita Hot Springs and Tomichi Dome area. The first phase consisted of mapping and profile dipole-dipole surveys and Schlumberger soundings. The mapping dipole survey consisted of 103 stations over a 3 by 4 mile area using a transmitting dipole 7,000 feet in length and an orthogonal pair of 300 foot dipoles at each station. Injected currents of 6-8 amperes and a filtering frequency of 3.0 Hz were used. The profile dipole survey used dipole with a length of 200 feet and transmitting-receiving station separations of 1-9 dipole lengths. An injected current of 5 amperes and 3.0 Hz frequency was used. The Schlumberger soundings involved a transmitting dipole with length ranging from 500-2,500 feet for a 100-foot receiving dipole to 2,500-15,000 feet for a 500-foot receiving dipole. Injected currents of 2-6 amperes were used with a frequency of 0.05 Hz read as an averaged DC-coupled signal by the receiver. The second phase consisted only of dipole-dipole mapping, with a transmitting dipole length of 7,000 feet and 132 stations spread over several areas of interest identified in Phase I. All stations used an orthogonal pair of 300-500 foot dipoles. Injected currents of 2-8 amperes were used with frequencies of either 0.1 or 0.3 Hz to eliminate induced coupling effects. All measurements were obtained using a GEOEX Mark 4 induced polarization-resistivity system [10].

The resistivity surveys identified a low-resistivity zone approximately 7 km by 3.5 km in area extending NW to SE between Waunita Hot Springs and Black Sage Pass. Apparent resistivities in the zone ranged from 33-300 ohm-meters, with the lowest readings in synclinal Mesozoic sediments 2-3 km west of Black Sage Pass. Henrichs Geoexploration Company [1981] interpreted this zone as a 50 ohm unit of Mesozoic sediments over a 2000 ohm Precambrian crystalline basement. Contour mapping also suggested a possible resistivity low beneath the southern flank of Tomichi Dome; however, poor access limited coverage beneath the dome itself [10]. It should be noted that these surveys were conducted prior to the advent of modern inversion techniques (and computing power) and, as such, a reanalysis of these data may prove fruitful in identifying or better characterizing anomalies potentially associated with geothermal reservoirs.
In addition to the geophysical data, in the summer of 1980, the Colorado Geologic Survey (CGS) analyzed 65 soil samples from the Waunita Hot Springs area for mercury concentrations as part of its geothermal resource assessment program. Samples were taken from a series of profile lines within an approximately 0.06 sq. mi area surrounding the hot springs (Figure 7). Additonally, several samples were taken approximately 1000 ft to the NE of the sample area to account for background soil mercury concentrations. Sample lines were designed to cross known nearby structural features as well as the near vicinity of the hot springs themselves. Soil mercury content ranged from 2-179 ppb in the analyzed sample, with a mean of 19.46 ppb and standard deviation of 31.76 ppb. Unfortunately, the survey results were inconclusive, with no identifiable pattern that would be indicative of thermal systems apart from those already known [11].
Eight thermal gradient boreholes were installed by AMAX Exploration, Inc. within a 3.6 km radius of Waunita Hot Springs, at an undetermined time prior to 1981 (Figure 8). All boreholes were drilled to 100 m depth except #3 and #7, which were completed to 88 and 82 m, respectively. Boring logs are of limited detail with regard to lithology, but generally indicate sequences of clay, shale, and sandstone, with some rhyolite in Boreholes #5 and #8 and schist in Borehole #6. The water table was encountered between 14 and 55 meters in most boreholes, though it is unknown if these boreholes were allowed to equilibrate first. Bottom hole temperatures for most ranged from 7.1ºC (in Borehole #7) to 14ºC (in Borehole #6); however, Borehole #3 exhibited an anomalously high bottom hole temperature of 47.5ºC, along with the highest calculated thermal gradients for any of the boreholes (Figure 9). Borehole #3 was the closest to the hot springs, located approximately 0.6 km to the southwest of the Upper Springs on the opposite side of County Road 887 [12].
Most boreholes exhibited significant anisotropy in thermal gradients, except Boreholes #1 and #6, for which differential thermal gradient data was not available, and Borehole #8, which was the furthest away (~3.6 km) from the hot springs, on the opposite side of Tomichi Dome. Again, Borehole #3 exhibited the greatest anisotropy, with a reported temperature gradient of 1,080ºC/km between 34-40 m depth, and gradients of 340ºC/km and 247ºC/km immediately above and below, respectively [12]. This anisotropy in thermal gradient argues for a hydrothermal system dominated by by fracture or similar preferential flow in these anomalously high zones. As such, the utility of these boreholes in assessing deeper crustal heat flow and potential reservoir temperatures is severely limited. Averaging the temperature gradient from the surface to the bottom of the boreholes may provide a more reasonable estimate of the overall thermal flow for the resource area. These values range from 25 to 62ºC/km for all the boreholes, with 25ºC/km representing the average thermal gradient of the continental crust. The boreholes which exhibited the lowest average gradient were several kilometers from the hot springs and up-dip. None of them penetrated the Dakota Sandstone [2].
Well Field Description
Well Field Information |
Development Area: | ||
Number of Production Wells: | 0 | |
Number of Injection Wells: | 0 | |
Number of Replacement Wells: | 0 | |
Average Temperature of Geofluid: | ||
Sanyal Classification (Wellhead): | ||
Reservoir Temp (Geothermometry): | 200°C 473.15 K 392 °F 851.67 °R |
|
Reservoir Temp (Measured): | ||
Sanyal Classification (Reservoir): | Moderate Temperature | [13] |
Depth to Top of Reservoir: | ||
Depth to Bottom of Reservoir: | ||
Average Depth to Reservoir: | ||
- "Local ranches generally have shallow water supply wells installed in the alluvium found along stream valleys '"`UNIQ--ref-00000020-QINU`"'. No other thermal, water supply, or oil and gas wells are documented or known to exist." cannot be used as a page name in this wiki.
- The given value was not understood.
Geology of the Area
Geologic Setting |
Tectonic Setting: | ||
Controlling Structure: | Intrusion Margins and Associated Fractures | [8] |
Topographic Features: | Lava Dome | [8] |
Brophy Model: | ||
Moeck-Beardsmore Play Type: | ||
Geologic Features |
Modern Geothermal Features: | ||
Relict Geothermal Features: | Hydrothermally Deposited Rock | [8] |
Volcanic Age: | Tertiary | [8] |
Host Rock Age: | ||
Host Rock Lithology: | ||
Cap Rock Age: | ||
Cap Rock Lithology: | ||
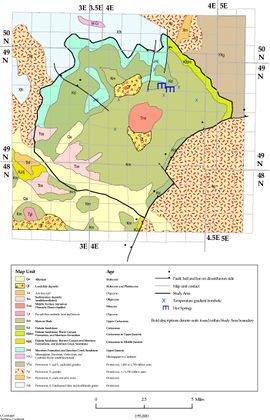
Regional Setting
Waunita Hot Springs resides in the southern Rocky Mountains, within the northeastern quadrant of an intermontane sedimentary basin resulting from a tectonic graben. To the north of the hot springs lies the Sawatch Range, which consist of complex batholitic cores of Precambrian to Tertiary granite exposed by the erosion of overlying units. Approximately 15 miles to the northeast of Waunita Hot Springs, the Mount Princeton, Mount Antero, Mount Shavano and their associated lesser peaks represent an early Tertiary batholith of granite with some andesite and quartz monzonite. Tomichi Dome, a medium-sized igneous body roughly 2 miles south of the hot springs, may represent an apophysis, or branch, of the Mount Princeton-Mount Antero-Mount Shavano batholith. To the southwest lie the San Juan Mountains, an approximately 15,000 sq. mi region of extrusive and intrusive Tertiary volcanics stretching from north-central New Mexico through southwestern Colorado. Radiometrically-dated Oligocene and Miocene-age ash-tuffs and other volcanics comprise the bulk of the San Juan complex, though younger volcanic, primarily basaltic, rocks are also common. Continual episodic volcanism from 40 to 0.5 million years before present is evident in the rock record for this region. Furthermore, a slow doming of the region during the last 2 million years may indicate continued activity in the region, with the possibility for a newly-emplaced batholith within a few miles of the surface [4].
Satellite photos of Colorado suggest that the Waunita Hot Springs lie on the intersection of at least three major linear features, namely the N-S Rio Grande rift zone, a northwest trending lineament toward the West Elk uplift, and a southwest trending lineament into the San Juan Mountains, with which the Crookton fault is presumed to be associated. It has been suggested that these lineaments may bely structural features which could function as conduits for magmatic intrusions or the flow of thermal waters [8].
Glaciation and erosion in the area have made significant impacts on topography. Quaternary landslide deposits and alluvial can be found at the base of slopes in small stream valleys in the area, with thicknesses generally estimated to be less than 500 feet [8][4][2].
The area around Waunita Hot Springs receives an average of 15 in. of precipitation per year, with closer to 20 in. falling on the peak of Tomichi Dome. Precipitation is mostly winter snowpack, with the remainder falling in summer thunderstorms. Three small perennial streams drain the area: Tomichi Creek, which runs 5 miles to the east of the hot springs before looping to the south and west, marking the boundary between the Sawatch Range and the San Juan Mountains; Cochetopa Creek, which joins Tomichi Creek approximately 15 miles to the west of the hot springs; and Quartz Creek, which runs toward the south, 8 miles west of Waunita Hot Springs. Additionally, Hot Springs Creek, a 15 mile long intermittent stream with its head at Waunita Pass, passes along the base of the Lower Springs before joining Tomichi Creek, with its flow being considerable augmented by the 1,000 gpm discharge of the Lower Springs [4].
Stratigraphy
Exposures of Precambrian rocks have been observed to the southeast, east, and northeast of the hot springs and Tomichi Dome (Figure 10).
Finely laminated muscovite schist with some quartz and biotite was noted to the north of the hot springs. The rock usually appears as gray or brownish-gray fine-grained schist with macroscopic muscovite, biotite and quartz. Variations include a dark quartz-biotite schist, light quartz-muscovite schist, and beds or lenses of pink to gray quartzite. Moderate to well-developed foliation is evident, with the schist grading into gneiss in some places [8].
Observed with the muscovite schist was a mildly gneissic granite. It is generally expressed as gray to brownish-gray with faint but distinct foliations. Narrow streaks, bands, or lenses of biotite and slightly smoky quartz define the foliation in an aggregate of light gray quartz, white to light gray feldspar, and some minute flakes of biotite. It is predominantly fine-grained, but can be locally medium- to coarse-grained. Reddish-brown, greenish-gray, and gray coloration appears on weathered surfaces [8].
Also near the muscovite schist and gneissic granite was a dark green diorite rich in hornblende. Typically the rock appears as massive and medium-grained, though it can be fine- to moderately coarse-grained as well. Greenish-gray and reddish brown coloration can appear on weathered surfaces. In hand specimens, feldspar and hornblende are the only macroscopically identifiable minerals [8].
In a quarry near Black Sage Pass, exposures of Pikes Peak granite can be found. The granite is usually pink from the many ½ to 1 inch long feldspar phenocrysts, and exhibits tiny grains of minimally-weathered black biotite. It can be coarse- to medium-grained. It can appear as gray to light gray where freshly broken [8].
Mesozoic sedimentary rocks from the Jurassic Morrison formation to Cretaceous Pierre shale unconformably overly the Precambrian basement and cover the area surrounding Waunita Hot Springs and Tomichi Dome with an estimated thickness of 2000 to 2600 feet [8].
The Jurassic Morrison formation, directly above the Precambrian unconformity, is comprised of interbedded yellowish to gray limestones, greenish or reddish shales, and fine- to medium-grained dirty white sandstones. The thickness is estimated to range from 250 to 375 feet, but the formation is easily weathered and has few outcrops in the area [8].
The Cretaceous Dakota sandstone, which overlies the Morrison formation, consists of generally medium-grained crossbedded quartzose sandstone or quartzite with a light-gray color, though it often appears reddish-brown to light brown where weathered. An up to 15-foot-thick conglomerate locally occupies the base of the formation and appears as lenses or thin beds in the lower layers. The upper part of the formation contains intercalated gray shale beds. The estimated total thickness of the Dakota is 150 to 200 feet, though the upper formation generally has poor exposure [8].
Above the Dakota lies the Benton formation. The lower Benton is a calcareous clay-shale with a light-gray color which weathers to a light yellow-brown. The upper Benton, alternatively referred to as the Carlile sandstone, and consists of two-foot thick alternating beds of fetid, calcareous sandstone with a chocolate-brown color and thin, dark-brown shale [8]. Overlying the Benton formation is the Niobrara limestone. It is appears as chalky limestone, white to light yellow-brown in color, which is parted by two to four inch thick beds of shale. On top of the Niobrara limestone, the Pierre shale covers a large portion of the surface surrounding Waunita Hot Springs and Tomichi Dome. It is a thick section of fissile shale, dark-brown to black in color, with locally interbedded sand, particularly near the top of the formation. In some layers, cone-in-cone structures have been observed. Collectively, the Niobrara limestone and Pierre shale make up the Mancos Group, which rests conformably on the Dakota [8].
A number or Tertiary igneous intrusives also exist in the area. The most prominent of these is the Tomichi Dome rhyolite. Although not radiometrically dated, the Tomichi Dome rocks are estimated to be of a similar age as the West Elk laccolith and older San Juan volcanics, roughly 30-40 million years before present. Tomichi Dome actually exhibits two distinct bodies of igneous rocks. One, a sill exposed in select locations around the dome, appears in hand specimens as a light-gray, aphanitic rock with platy fracture. At the microscopic scale, phenocrysts of feldspar, biotite, and quartz can be seen in a glassy matrix. The close resemblance of this sill to the main igneous mass seen at Tomichi Dome suggests that the two are of a similar origin. The main igneous body comprises the top of Tomichi Dome, covering roughly three square miles with a thickness of greater than 1800 feet. It is everywhere light-gray and fine-grained, but in its lowest exposed depths exhibits a tuff-like texture with a thickness of approximately 700 feet, above which it adopts a variolitic-like texture with a thickness of close to 900 feet. The topmost portion of the dome is largely equigranular. This sequence is best exposed on the northern slope of the dome, toward the hot springs [8].
The topmost facies exhibits a fine-grained to stony texture, with some phenocrysts of biotite, feldspar, and quartz visible at the macroscopic scale. At the microscopic scale, it consists of 15 to 30 percent glass matrix containing irregulary-rounded areas suggestive of spherulites. Crystals of feldspar, biotite, and quartz make up the majority of the remaining composition, with quartz averaging 7 to 10 percent, brown biotite averaging 2 to 10 percent, and roughly equal amounts of orthoclase and oligoclase feldspar, indicating a composition similar to quartz monzonite intrusions seen in many parts of central Colorado. Some topaz and magnetite can be seen in thin section. Garnet can also be found in the more tuff-like facies. Although not visible in thin section, small amounts of apatite, hornblende, ilmenite, and titanite were identified, though in much smaller quantities than the topaz, magnetite, and garnet [8].
The variolitic-like facies is mineralogically identical with the topmost facies. Upon weathering, the spherules in the middle member, ranging from less than 0.05 to 0.5 inches in diameter, begin to show as light-gray area surrounded by discolored brownish matrix. At the microscopic scale, true variolitic texture is not apparent [8].
The basal, tuffaceous facies also bears a similar composition to the upper facies. At the macroscale, it exhibits up to inch-long sub-angular pieces with weathering, set in a fine aggregate. The clastic texture suggests a highly silicified tuff or breccia thrown in ash and pyroclastic eruptions and subsequently cemented with silica. This interpretation is supported at the microscopic scale as well [8].
In addition to the rhyolite of Tomichi Dome, a Tertiary monzonitic dike of gray to olive-green rock with prominent altered phenocrysts of white feldspar can be seen to the east of Tomichi Dome, where it cuts through the Late Cretaceous Mancos sediments. Additionally, pyritic quartz veins with associated altered sedimentary rocks can be found in the area [8].
Structure
Structure around Waunita Hot Springs is dominated by the Crookton fault, the major fault in the area, and the intrusion of Tomichi Dome. A minor local regional southeast dip in sediments ranges from 6° to 40° with a strike to the northeast. Sediments surrounding Tomichi Dome are generally monoclinal [8].
The nearby Crookton reverse fault is 30 miles in length and trends NNE-SSW. The fault runs from Pitkin, 15 miles north of the hot springs, through Horn Gulch, 3 miles east of the springs, and further southward to a point 15 miles southeast of Waunita Hot Springs. The dip is unknown, but cannot be less than 45° based upon its interaction with topography, with the eastern block being the up-thrown side. Observed displacement and drag on adjacent beds indicate significant movement, which has led others to refer to it as a “thrust” fault despite its inferred dip. Some amount of oblique slip is also inferred, based on the geometry of exposed sedimentary layers, with the eastern side of the fault moving somewhat northward relative to the western side Overturned beds of Dakota sandstone and Benton formation exist along the Crookton fault, which appears to thrust Precambrian granite over the Mesozoic sediments to the northwest. Midway along its trace, the fault brings Morrison shaly sandstones and shales to the surface, which crop out between the Dakota and Precambrian granite. North of this, the Dakota outcrops with vertical beds and ripple marks as a steep cliff, almost a quartzite in composition. [8].
Due to the Crookton fault, the sediments which underlie Tomichi Dome have been steeply up-turned where they approach the fault. Abrupt and irregular minor folds have formed as a result, particularly in the plastic Pierre shale. The combined effects of the southeastward regional dip and the imposed northwest dip from the Crookton fault have created a basin-like structure, with the lowest structural point about a mile to the southwest of Tomichi Dome [8].
In addition to the large Crookton fault, smaller radial and concentric normal faults have formed around Tomichi Dome, likely resulting from the intrusion, and ultimate eruption, of the magmas which formed Tomichi Dome (Figure 11). Throw on some of these faults can be up to several hundred feet. As such, these faults may enable deep flow around any potential geothermal cells, particularly given their inferred volcanic origin [8].
Tectonic and intrusive activity has likely spurred fracturing in the Dakota sandstone, which has mostly vertical dips and strikes from N-S to E-W. Despite their abundance and density, the joints are not assumed to have continuity with depth, though the fault system could potentially extend discontinuous jointing to the basement, which would add significantly to the porosity and permeability of the system barring the precipitation of secondary deposits within the fractures. Limonite observed on some joints could be indicative of hot waters which mobilized pyrite and then reprecipitated it as limonite [8].
Hydrothermal System
- "Two groups of hot springs, the Upper and Lower Springs, are at expressed at the surface at Waunita Hot Springs. The Upper Springs, consisting of four springs, issue from the fault-exposed contact between the Dakota sandstone and the overlying shales of the Mancos Group, which act as confining unit '"`UNIQ--ref-00000040-QINU`"''"`UNIQ--ref-00000041-QINU`"'. Temperatures of 57°C to 78°C have been reported for the Upper Springs, with discharges ranging from 171 to 1000 L/min '"`UNIQ--ref-00000042-QINU`"'. The maximum temperature and discharge from the Upper Springs represent a nearly 200 million g-cal/min flow of heat from the springs '"`UNIQ--ref-00000043-QINU`"'. The Lower Springs consist of three sub-groups of springs issuing from a fault zone within the Dakota sandstone '"`UNIQ--ref-00000044-QINU`"''"`UNIQ--ref-00000045-QINU`"'. The Lower Springs have a slightly lower average temperature, with recorded values ranging from 63°C to 70°C for an associated discharge of 156 to 300 L/min '"`UNIQ--ref-00000046-QINU`"'.
Other hot springs also exist on the opposite side of the Continental Divide near Salida (Poncha Springs) and Mt. Princeton (Hortense Hot Spring). Poncha Springs is on average slightly cooler than Waunita, with temperatures ranging from 56°C to 70°C with discharges ranging from less than 8 L/min to 760 L/min. However, the Hortense Hot Spring at Mt. Princeton is the hottest in the state, with a recorded temperature of 83°C, though discharge is only a meager 74 L/min '"`UNIQ--ref-00000047-QINU`"'. The proximity of these hot springs to Waunita Hot Springs and the batholitic complex of Mount Princeton, Mount Antero, and Mount Shavano supports an intrusive origin for the geothermal heat expressed at Waunita Hot Springs '"`UNIQ--ref-00000048-QINU`"'.
The Dakota sandstone is the presumed hydrothermal conduit to the surface '"`UNIQ--ref-00000049-QINU`"'. Based on porosity and permeability analyses of surface samples from the Dakota, the unit has been shown to vary in horizontal permeability from 0.1 to 66 Millidarcys and in porosity from 1.1 to 10.5 percent '"`UNIQ--ref-0000004A-QINU`"'. This substantial variability in permeability and porosity likely indicates the predominance of fractures in the flow patterns through the Dakota. Geothermal waters are therefore inferred to flow through the basal conglomerate, upper and lower contacts, and fracture and shear networks rathern than through the matrix of the Dakota '"`UNIQ--ref-0000004B-QINU`"'. Indeed, the Dakota is shown to be well-fractured near fault zones and local Tertiary intrusions '"`UNIQ--ref-0000004C-QINU`"'. The thick shales which bound the Dakota at its upper and lower contacts, the Mancos Group and Morrison formation, respectively, are poor hydrologic conductors and effectively isolate any geothermal waters moving through the Dakota, except where local fracturing permits fluid egress. Due to regional and local dipping and faulting, the depth to the Dakota sandstone can be highly variable and would merit concerted study prior to selecting a production well site '"`UNIQ--ref-0000004D-QINU`"'.
The geochemical work of Carpenter [1981] and Dellechaie [1981] provides some evidence for the existence of a vapor/steam-dominated system at depth. This conclusion is somewhat questionable, however, as significant advancements have been made in the field since these samples were collected and analyzed in 1974. Furthermore, little is known about the flow path of the hydrothermal system at depth; an extensive, detailed geochemical study would be necessary to confirm the presence of a vapor/steam-dominated system. Even should such a system be proven to exist, it may still be uneconomical to develop the geothermal resource at such a depth '"`UNIQ--ref-0000004E-QINU`"'.
Since the reservoir and heat source for this system have yet to be constrained, it is difficult to assess potential sources of recharge to the system. No isotopic studies which might indicate origin or age of the thermal waters issuing from the springs have been conducted. It is likely that faulting and fracturing in the area plays a significant role in conducting meteoric surface waters to the geothermal reservoir, be it shallow or deep '"`UNIQ--ref-0000004F-QINU`"'. Alluvium along creek channels may assist infiltration and recharge into fracture zones feeding deep or shallow systems '"`UNIQ--ref-00000050-QINU`"'. If the geothermal reservoir is heated by Tertiary intrusives, then recharge is probably confined to the local area, and geothermal waters are most likely sourced from the Dakota sandstone. If, however, a deep, steam-dominated system located in the metamorphic regime of the Proterozoic basement is the source of the thermal waters issuing from the hot springs, as supported by the geochemical analyses of Carpenter [1981]'"`UNIQ--ref-00000051-QINU`"' and Dellechaie [1981]'"`UNIQ--ref-00000052-QINU`"', then recharge is likely regional with faults and fractures in the basement conveying water to the reservoir '"`UNIQ--ref-00000053-QINU`"'." cannot be used as a page name in this wiki. - The given value was not understood.
Other hot springs also exist on the opposite side of the Continental Divide near Salida (Poncha Springs) and Mt. Princeton (Hortense Hot Spring). Poncha Springs is on average slightly cooler than Waunita, with temperatures ranging from 56°C to 70°C with discharges ranging from less than 8 L/min to 760 L/min. However, the Hortense Hot Spring at Mt. Princeton is the hottest in the state, with a recorded temperature of 83°C, though discharge is only a meager 74 L/min [16]. The proximity of these hot springs to Waunita Hot Springs and the batholitic complex of Mount Princeton, Mount Antero, and Mount Shavano supports an intrusive origin for the geothermal heat expressed at Waunita Hot Springs [4].
The Dakota sandstone is the presumed hydrothermal conduit to the surface [2]. Based on porosity and permeability analyses of surface samples from the Dakota, the unit has been shown to vary in horizontal permeability from 0.1 to 66 Millidarcys and in porosity from 1.1 to 10.5 percent [8]. This substantial variability in permeability and porosity likely indicates the predominance of fractures in the flow patterns through the Dakota. Geothermal waters are therefore inferred to flow through the basal conglomerate, upper and lower contacts, and fracture and shear networks rathern than through the matrix of the Dakota [2]. Indeed, the Dakota is shown to be well-fractured near fault zones and local Tertiary intrusions [8]. The thick shales which bound the Dakota at its upper and lower contacts, the Mancos Group and Morrison formation, respectively, are poor hydrologic conductors and effectively isolate any geothermal waters moving through the Dakota, except where local fracturing permits fluid egress. Due to regional and local dipping and faulting, the depth to the Dakota sandstone can be highly variable and would merit concerted study prior to selecting a production well site [2].
The geochemical work of Carpenter [1981] and Dellechaie [1981] provides some evidence for the existence of a vapor/steam-dominated system at depth. This conclusion is somewhat questionable, however, as significant advancements have been made in the field since these samples were collected and analyzed in 1974. Furthermore, little is known about the flow path of the hydrothermal system at depth; an extensive, detailed geochemical study would be necessary to confirm the presence of a vapor/steam-dominated system. Even should such a system be proven to exist, it may still be uneconomical to develop the geothermal resource at such a depth [2].
Since the reservoir and heat source for this system have yet to be constrained, it is difficult to assess potential sources of recharge to the system. No isotopic studies which might indicate origin or age of the thermal waters issuing from the springs have been conducted. It is likely that faulting and fracturing in the area plays a significant role in conducting meteoric surface waters to the geothermal reservoir, be it shallow or deep [2]. Alluvium along creek channels may assist infiltration and recharge into fracture zones feeding deep or shallow systems [4]. If the geothermal reservoir is heated by Tertiary intrusives, then recharge is probably confined to the local area, and geothermal waters are most likely sourced from the Dakota sandstone. If, however, a deep, steam-dominated system located in the metamorphic regime of the Proterozoic basement is the source of the thermal waters issuing from the hot springs, as supported by the geochemical analyses of Carpenter [1981][17] and Dellechaie [1981][15], then recharge is likely regional with faults and fractures in the basement conveying water to the reservoir [2].
Heat Source
- "At present, no definitive heat source has been identified for the thermal waters issuing from Waunita Hot Springs. Most interpretations infer an intrusive magmatic body, related to either the Sawatch Range or the Rio Grande Rift, at a depth of a few miles beneath the resource area '"`UNIQ--ref-00000054-QINU`"''"`UNIQ--ref-00000055-QINU`"''"`UNIQ--ref-00000056-QINU`"'. It is also possible that cooling, near-surface Tertiary intrusives related to Tomichi Dome are supplying the heat. Extrapolated average geothermal gradients from boreholes would indicate a moderate reservoir temperature of up to 120°C at a depth of 5,000 ft, suitable for binary geothermal power generation '"`UNIQ--ref-00000057-QINU`"'. Geothermometry studies conducted on water from the hot springs by Barrett and Pearl [1976]'"`UNIQ--ref-00000058-QINU`"' and Pearl [1979]'"`UNIQ--ref-00000059-QINU`"' indicate an equilbrium reservoir temperature of 175°C to 225°C at its deepest portion; using the geothermal gradients extrapolated from the boreholes, these temperatures would occur at a depth of approximately 5 miles, a seemingly unreasonable depth for the reservoir which indicates the potential inaccuracy of gradients calculated from the thermal boreholes '"`UNIQ--ref-0000005A-QINU`"'." cannot be used as a page name in this wiki.
- The given value was not understood.
Geofluid Geochemistry
Geochemistry |
Salinity (low): | 528 | [13] |
Salinity (high): | 613 | [13] |
Salinity (average): | 564 | [13] |
Brine Constituents: | B, Ca, Cl, F, Fe, Li, Mg, Mn, K, Na, NH3, H2S, PO4, SiO2, SO4, CO3, HCO3 | [13][15] |
Water Resistivity: | 850 | [17] |
The study by Barrett and Pearl [1976][14] collected and analyzed five water samples from the Upper Springs and four samples from the Lower Springs between 1975 and 1976. Samples were analyzed for major ion and metal concentrations as well as alkalinity, pH, hardness, specific conductance, and total dissolved solids.
The geothermometry study by Pearl [1979][13] provided quartz-silica, Na-K, and Na-K-Ca geothermometry estimates and mixing model analyses based on the samples collected by Barret and Pearl [1976][14]. Close agreement was achieved between the mixing model and the Na-K-Ca model estimates, indicating a reservoir temperature of 175°C to 225°C and heat content of 0.0606 quadrillion BTU's [13].
In July 1974, Carpenter [1981][17] collected 21 water samples in sets of 3 from 7 different sites around Waunita Hot Springs, including the hot springs, two cold springs, and three surface water sites along Hot Springs Creek. Each sample set was analyzed for silica, sodium, potassium, calcium, magnesium, manganese, zinc, fluorine, chlorine, pH, and conductivity. Low concentrations of chlorine, calcium, and magnesium from the hot spring samples were interpreted as suggestive of a vapor-dominated system at depth, since higher concentrations of these elements would be present in meteoric or magmatic waters which had not undergone a phase change.
Dellechaie [1981][15] collected water samples from two of the Upper Springs on April 12, 1975, in order to compare their geochemistry to that documented at Hortense Hot Spring in Mt. Princeton, Colorado and near the Geysers in Lake County, California. The Waunita Hot Springs samples exhibited similar sulfate, bicarbonate, potassium, lithium, boron, chlorine, silica, and total dissolved solids concentrations to springs at the Geysers, CA. Waunita Hot Springs samples differed from the Geysers samples in fluorine, sodium, and ammonia concentrations as well as chlorine/sulfate and chlorine/fluorine ratios. Furthermore, inferred mineral suites at reservoir depths also differed between the two locales.
NEPA-Related Analyses (0)
Below is a list of NEPA-related analyses that have been conducted in the area - and logged on OpenEI. To add an additional NEPA-related analysis, see the NEPA Database.
CSV No NEPA-related documents listed.
Exploration Activities (8)
Below is a list of Exploration that have been conducted in the area - and cataloged on OpenEI.
Add a new Exploration Activity
References
- ↑ 1.0 1.1 1.2 U.S. Geological Survey. 2008. Assessment of Moderate- and High-Temperature Geothermal Resources of the United States. USA: U.S. Geological Survey. Report No.: Fact Sheet 2008-3082.
- ↑ 2.00 2.01 2.02 2.03 2.04 2.05 2.06 2.07 2.08 2.09 2.10 2.11 2.12 2.13 2.14 2.15 A. M. Elser, A. L. Robbins, D. P. Stilwell (U.S. Department of the Interior Bureau of Land Management). 2010. Geothermal Resource Reasonably Foreseeable Development Scenario for Electrical Generation, Tomichi Dome and Surrounding Area, Grand Mesa-Uncompahgre-Gunnison National Forest, Colorado. Cheyenne, Wyoming: U.S. Department of the Interior Bureau of Land Management.
- ↑ 3.0 3.1 Www.waunita.com [Internet]. [cited 2014/05/08]. Available from: http://waunita.com/
- ↑ 4.00 4.01 4.02 4.03 4.04 4.05 4.06 4.07 4.08 4.09 4.10 4.11 GeothermEx (Colorado Geological Survey in Cooperation with the U.S. Department of Energy). 1981. Waunita Hot Springs, Colorado Geothermal Prospect Reconaissance. Denver, Colorado: Colorado Geological Survey in Cooperation with the U.S. Department of Energy. Report No.: Special Publication 16.
- ↑ http://www.census.gov/#
- ↑ 6.0 6.1 J. Behrendt, L. Bajwa. Bouguer gravity map. [Map]. Denver, Colorado. U.S. Geological Survey. (!) . Black & White. Scale 1:500,000.
- ↑ 7.0 7.1 Zietz and Kirby. Aeromagnetic map. [Map]. Denver, Colorado. U.S. Geological Survey. 1972. Scale 1:500,000.
- ↑ 8.00 8.01 8.02 8.03 8.04 8.05 8.06 8.07 8.08 8.09 8.10 8.11 8.12 8.13 8.14 8.15 8.16 8.17 8.18 8.19 8.20 8.21 8.22 8.23 8.24 8.25 8.26 8.27 8.28 8.29 K. W. Nickerson and Associates (Colorado Geological Survey in Cooperation with the U.S. Department of Energ). 1981. Report on Waunita Hot Springs Project, Gunnison County, Colorad. Denver, Colorado: Colorado Geological Survey in Cooperation with the U.S. Department of Energ. Report No.: Special Publication 16.
- ↑ A. L. Lange (Colorado Geological Survey in Cooperation with the U.S. Department of Energy). 1981. The Geophysical Environment Around Waunita Hot Springs. Denver, Colorado: Colorado Geological Survey in Cooperation with the U.S. Department of Energy. Report No.: Special Publication 16.
- ↑ 10.0 10.1 Heinrichs Geoexploration Company (Colorado Geological Survey in Cooperation with the U.S. Department of Energy). 1981. Geothermal resistivity resource evaluation survey Waunita Hot Springs project, Gunnison County, Colorado. Denver, Colorado: Colorado Geological Survey in Cooperation with the U.S. Department of Energy.
- ↑ 11.0 11.1 C. D. Ringrose, R. H. Pearl (Colorado Geological Survey in Cooperation with the U.S. Department of Energy). 1981. Soil mercury investigations, Waunita Hot Springs. Denver, Colorado: Colorado Geological Survey in Cooperation with the U.S. Department of Energy. Report No.: Special Publication 16.
- ↑ 12.0 12.1 12.2 T. G. Zacharakis (Colorado Geological Survey in Cooperation with the U.S. Department of Energy). 1981. Temperature, heat flow maps and temperature gradient holes. Denver, Colorado: Colorado Geological Survey in Cooperation with the U.S. Department of Energy. Report No.: Special Publication 16.
- ↑ 13.0 13.1 13.2 13.3 13.4 13.5 13.6 13.7 13.8 R.H. Pearl (Colorado Geological Survey in Cooperation with the U.S. Department of Energy). 1979. Colorado's hydrothermal Resource Base -- An Assessment. Denver, colorado: Colorado Geological Survey in Cooperation with the U.S. Department of Energy. Report No.: Resource Ser. 6.
- ↑ 14.0 14.1 14.2 14.3 14.4 14.5 James K.Barrett and Richard Howard Pearl. 1976. Hydrological Data of Thermal Springs and Well in Colorado. Denver, Colorado: Colorado Geological Survey Department of Natural Resource. Report No.: Information Series 6.
- ↑ 15.0 15.1 15.2 15.3 15.4 15.5 15.6 F. Dellechaie (Colorado Geological Survey in Cooperation with the U.S. Department of Energy). 1981. A hydrogeochemical comparison of the Waunita Hot Springs, Hortense, Castle Rock and Anderson Hot Springs. Denver, Colorado: Colorado Geological Survey in Cooperation with the U.S. Department of Energy. Report No.: Special Publication 16.
- ↑ 16.0 16.1 16.2 James A. Cappa and H. Thomas Hemborg (Colorado Geological Survey Department of Natural Resource). 1995. 1992-1993 Low-Temperature Geothermal Assessment Program, Colorado. Denver, Colorado: Colorado Geological Survey Department of Natural Resource. Report No.: Open File Report 95-1.
- ↑ 17.0 17.1 17.2 17.3 17.4 R. H. Carpenter (Colorado Geological Survey in Cooperation with the U.S. Department of Energy). 1981. Interpretation of Water Sample Analysis, Waunita Hot Spring Project, Gunnison County, Colorado. Denver, Colorado: Colorado Geological Survey in Cooperation with the U.S. Department of Energy. Report No.: Special Publication 16.
List of existing Geothermal Resource Areas.
Some of the content on this page was part of a case study conducted by: CSM 2014
{{}}